Top 10 discoveries about ancient people from DNA in 2024
New resolution is emerging of some events in ancient human populations, and a clearer view of some parts of the genome.
Rapid natural selection across the last 5000 years, a complex speciation to kick off the hominin lineage, and a single pulse of Neandertal ancestry into recent people around 47,000 years ago. Those are some of the big stories coming from DNA this year.
If you're thinking these stories sound like news from more than a decade ago, you're not alone. What's changed?
One big change, noted in my roundup last year, is the fast-growing sample of genome data from ancient people—now more than 10,000 individuals. That ancient sample improves on data from living people in some important ways. Today's people share genealogical ties that intertwine within a few hundred years as we look backward in time, and those ties ultimately limit the evidence that living people carry about our past. Meanwhile, the important events in our evolution are related to DNA through probability distributions that tend to smear together over thousands of generations. Ancient DNA can open the aperture, giving a closer view of events near the time they happened—key in this year's findings to the timing of Neandertal mixture and pace of recent natural selection.
Another change is the growing evidence of genetic variation in living nonhuman primates. Three of the insights I highlight this year owe directly to this growing record of DNA variation outside our species, which is enabling a clearer view of structural genome evolution in our ancestors.
Finally, some of the biggest advances in our evidence have come from human geneticists' ongoing work to increase the inclusion of groups who have long been underrepresented in genetic studies. Working with descendant communities to look at skeletal remains of their ancestors has been one important part of this work. Even more significant has been building resources for longitudinal and cross-sectional studies of health in nations that are developing greater capacity in genomics. Both approaches are providing a groundwork for new syntheses of population history, here seen in new DNA work from Rapa Nui, Papua New Guinea, and India.
So I've selected ten studies that represent these varied sources of new information. Several of them are nailing down ideas that were proposed early in this century—including some ideas from my own research. Others are pushing the boundaries of what we can say about phenotype evolution in living and ancient people. Together they give a picture of the way this area of research is changing our view of the human past.
No Rapa Nui population collapse
Twenty years ago, Jared Diamond's book Collapse: How Societies Choose to Fail or Succeed was published. The main idea of the book was that ancient human societies succumbed to disasters of their own making, as they failed to plan for the long-term consequences of decisions that had short-term benefits. One of the examples that Diamond drew upon was the history of Rapa Nui, often known as Easter Island. This island, some 2200 miles from the western coast of South America, was first inhabited by people who sailed there from Polynesia around 800 years ago. In Diamond's telling, the population of Rapa Nui rapidly grew, outstripping local resources and leading to deforestation and extinction of local plants and animals. War, cannibalism, and construction of the massive statues known as moai followed, accompanying an inevitable population collapse.
But archaeologists, led by Terry Hunt and Carl Lipo, quickly pointed out inconsistencies in this storyline of “ecological suicide”. Human arrival on the island did result in deforestation and other ecological impacts, exacerbated by the introduction of rats, but there was little evidence of a chain reaction of warfare and famine.
Last year, ancient DNA evidence made a contribution to better understanding the founding of Rapa Nui, its subsequent interactions with other populations, and its population history. Victor Moreno-Mayar and collaborators sampled DNA from early Rapa Nui skeletal remains held at the Musée de l'Homme in Paris, documenting the contributions of Polynesian and South American ancestors of the island's population prior to European colonial contact. The genealogies of the ancient individuals were consistent with steady population growth, even during the period of supposed collapse.
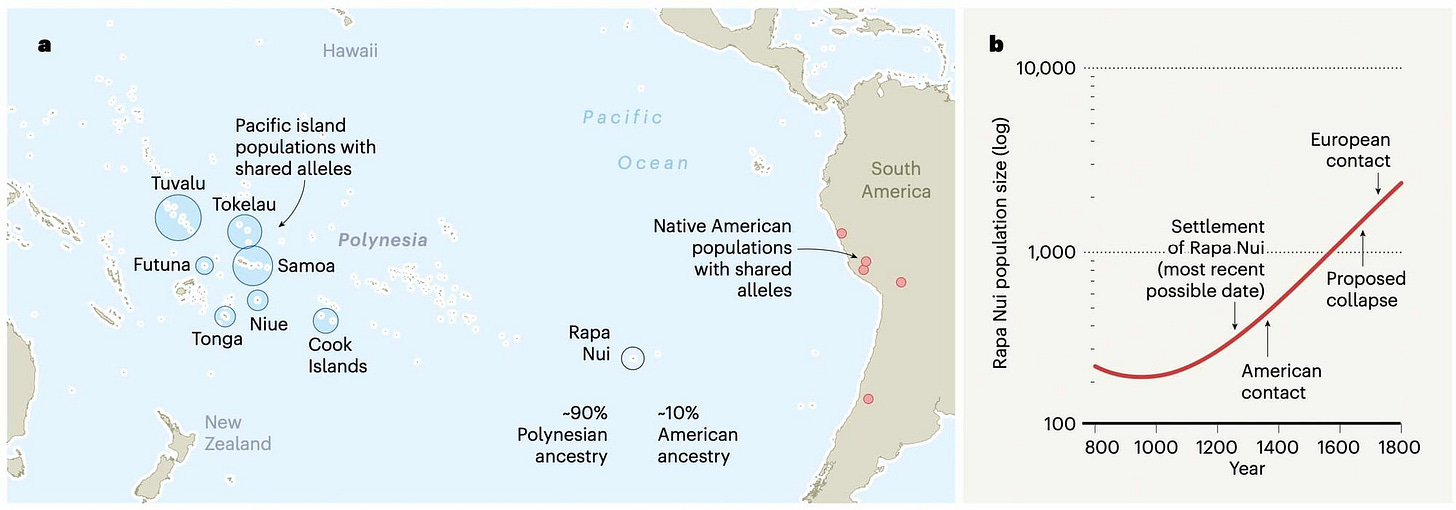
The results show that whatever impact humans had upon the island's ecology, the human population did not undergo a rapid boom and crash, but instead slow and steady growth. As they conclude, “our results support that the Rapanui population was resilient despite a changing environment.” In a final passage of the article, the researchers report within their article upon their consultations with Rapa Nui community representatives, who seek the return of the skeletal remains held in France.
Natural selection on the rise
When geneticists can sample enough ancient individuals, DNA enables them to construct a time sequence of allele frequencies. Such data are a movie of evolution. When alleles that were connected with phenotypic traits changed rapidly in frequency, differently from most of the genome, natural selection was the likely cause. Over the past few years samples of ancient genomes have become numerous enough to observe the effects of natural selection in some times and places.
This year several research studies have expanded our view of natural selection in the northern and western Eurasian populations where ancient DNA evidence is best represented. In January, a study by Evan Irving-Pease and coworkers started from UK Biobank data to trace local ancestry tracts linked to phenotypes. By examining these haplotypes in genomes from the last 10,000 years or more, the study was able to show associations between ancestry and phenotypes. The approach led to the identification of “ancestry-stratified” evidence of selection on alleles associated with phenotypes, from lactase persistence and skin pigmentation to fat metabolism.
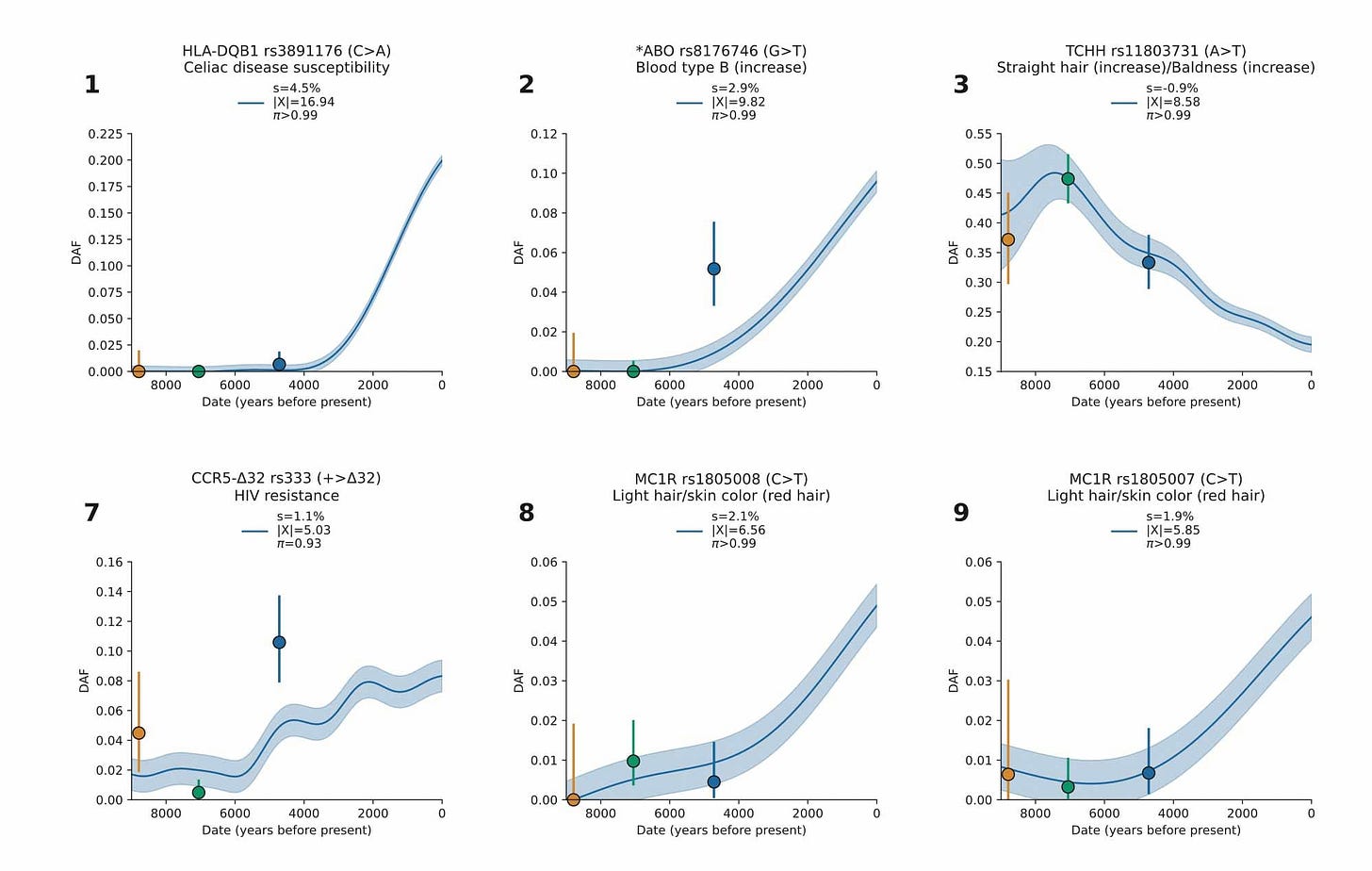
A second approach was taken by Ali Akbari and coworkers from David Reich's research group. They started with the largest sample of ancient DNA yet amassed covering more than 8000 people from the last 14000 years. Looking at the time series of DNA samples, they could test for frequency changes that stand out from the pattern of population structure that linked European populations across this time period. They confirmed hundreds of candidates for natural selection, affecting many traits.
I am very excited to see this area of research developing. My own work in 2007 showed that natural selection had likely accelerated in rate during the last few thousand years, due to larger population sizes and cultural changes that imposed new opportunities and costs for genetic variants. The new study by Akbari and coworkers is one of the first with enough statistical power to test the idea directly with ancient DNA. The data enabled the team to make some estimates of how the power of their approach would increase with larger samples, confirming that the current picture is likely to underrepresent the amount of selection that happened in this region in the past.
The evolution of the Major Histocompatibility Complex
The most diverse class of genes in the human genome belong to families known as the Human Leukocyte Antigen (HLA) system. The function of these genes is to bind to peptides from pathogens, enabling immune T cells to recognize them. In other vertebrates these families are known as the Major Histocompatibility Complex (MHC). Many of these genes have long-lasting trans-specific polymorphisms, meaning that a human allele may be closer to an allele in a macaque than to other alleles of the same gene in humans. These polymorphisms have been maintained by balancing selection from pathogens and parasites, sometimes for tens of millions of years. The great diversity of these genes has made them challenging to study.
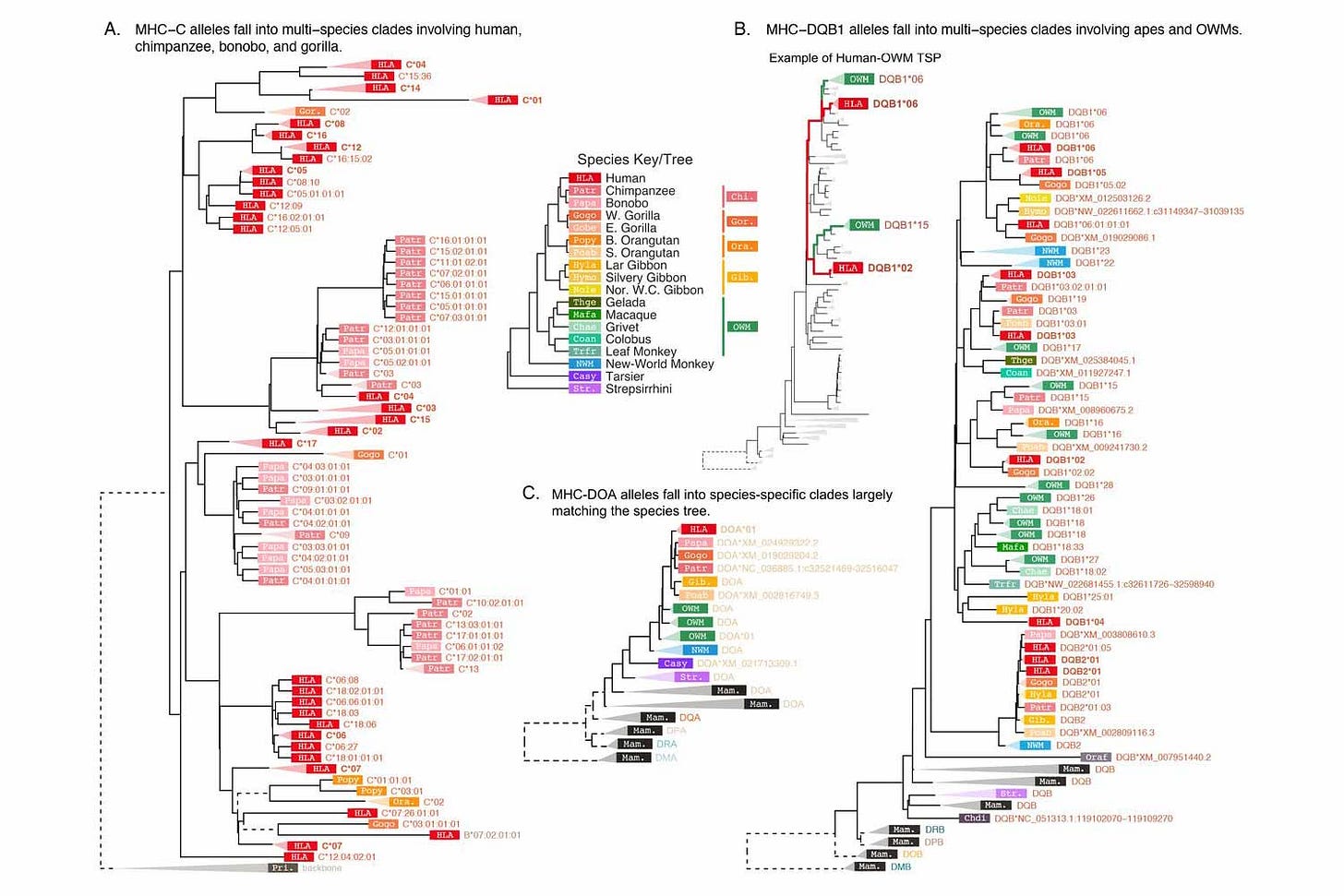
A pair of preprints from Alyssa Lyn Fortier and Jonathan Pritchard this year adds new resolution to the tree of MHC evolution in humans and other primates. In one study, they examine the level of trans-specific polymorphism, documenting human polymorphisms shared with chimpanzees, with gorillas or orangutans, and—in the case of DQB1 alleles—shared with cercopithecoid monkeys after more than 30 million years. The sister study shows the expansion, rearrangements, and formation of pseudogenes across primate lineages, with keys to understand the sequence of changes.
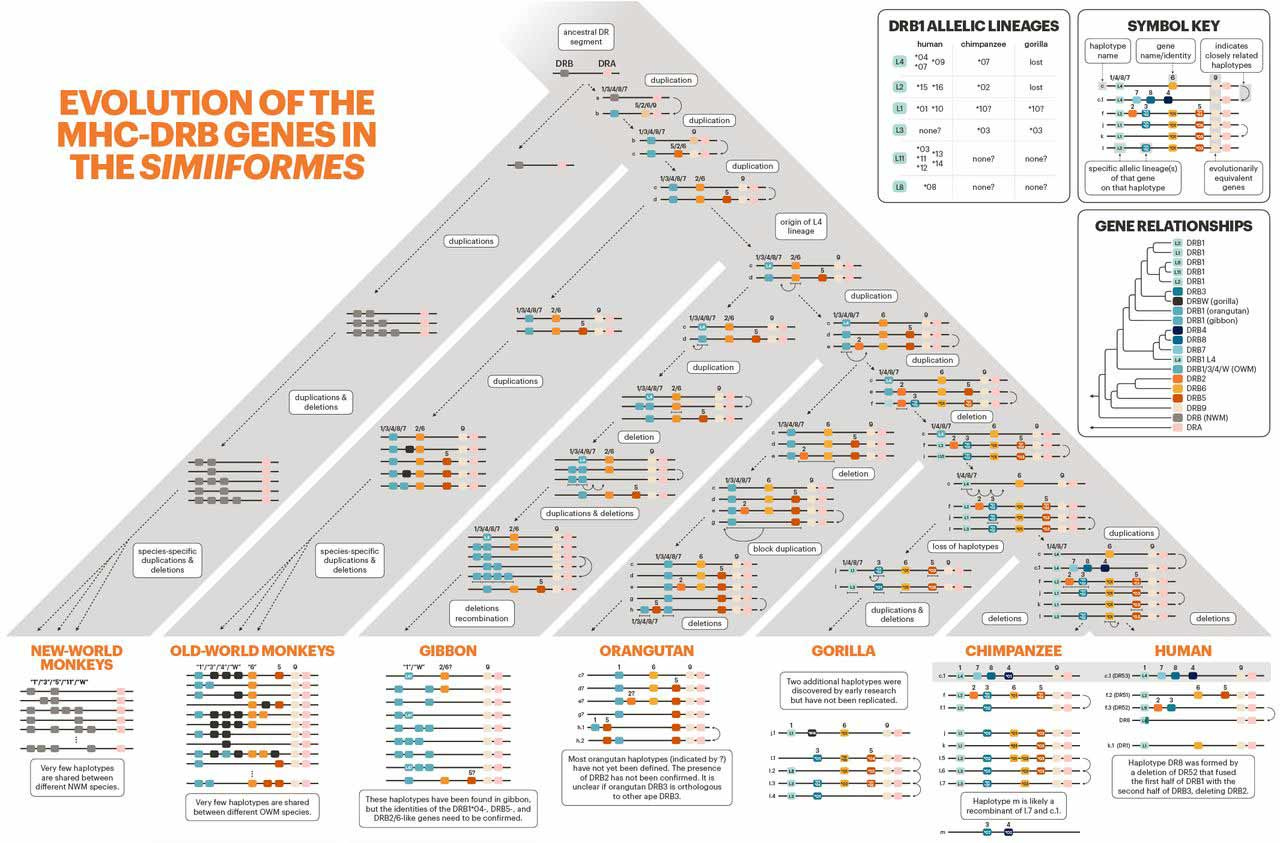
Trans-specific MHC polymorphisms in humans and other great apes were also shared in Australopithecus, and are one of the few ways that we may learn about the pathogens and parasites faced by these early human ancestors. This history is the foundation underlying the introgression of Neandertal and Denisovan HLA alleles into recent humans. One of the more interesting findings from the study by Akbari and coworkers, mentioned above, is the finding of strong recent selection on some HLA alleles during the last 5000 years. These genes continue to be extremely important to human health and disease.
Hominins and starch go way back
People today carry duplications of the genes for the enzyme, amylase, that breaks down starch. Amylase is produced in the pancreas through expression of the gene AMY2 and in the salivary glands through expression of AMY1, and both vary in copy numbers in different people's genomes. Populations with a long history of agriculture carry more copies of amylase genes. This covariation has been suggested as an adaptation to agricultural diets with starch-rich foods like grains and tubers. But it's hard to study the evolution of copy number variation, especially because aligning regions with duplications and gene conversions in ancient DNA data is challenging.
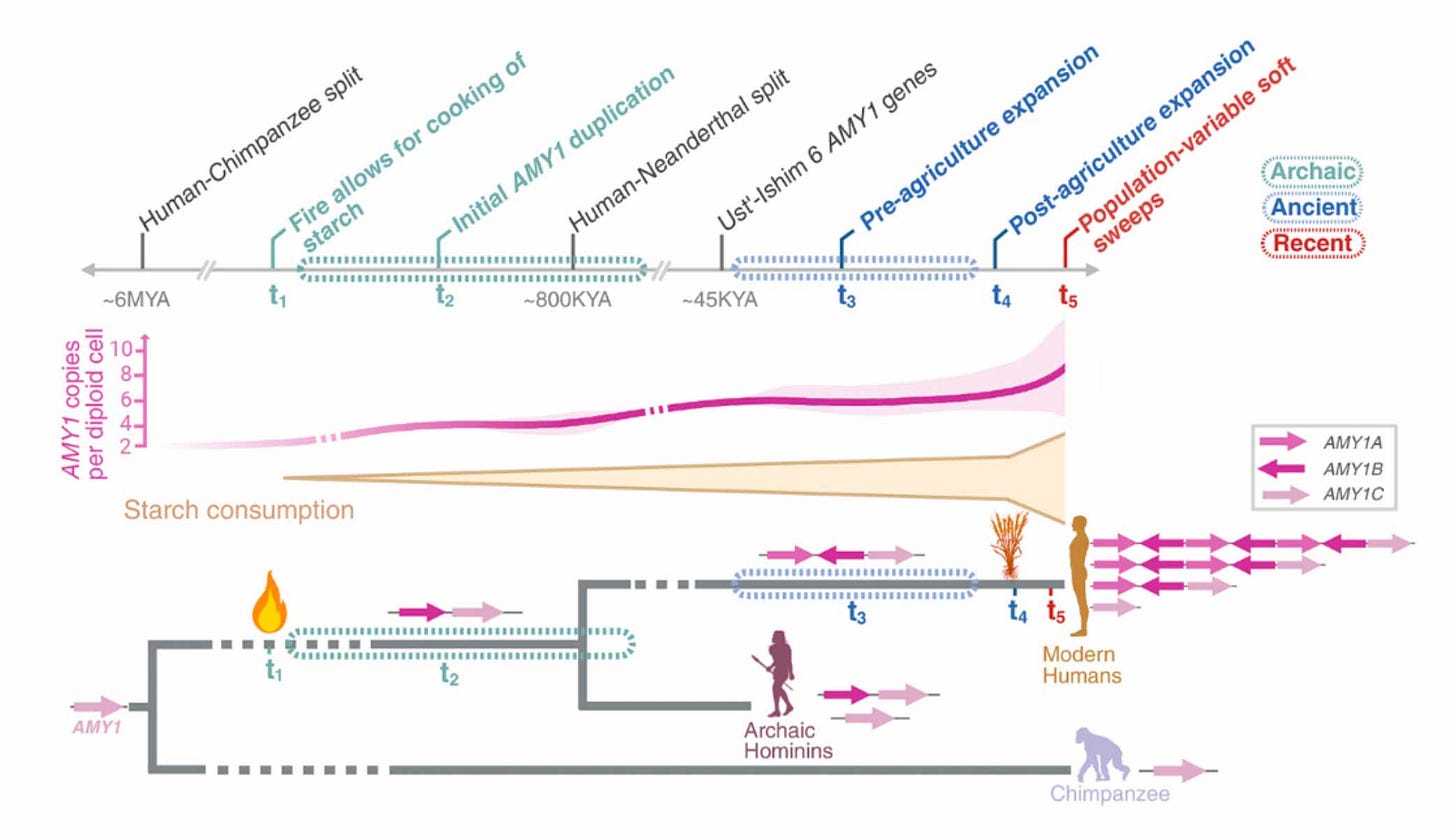
In a new study published this fall, Feyza Yılmaz and collaborators characterized more than 30 haplotypes of the gene region of chromosome 1 with AMY1, AMY2A, and AMY2B genes in humans around the world. Applying their findings to ancient DNA data, they were able to examine the copy number of AMY1 in some past populations, confirming a rapid increase in copy number in agricultural groups during the last 4000 years.
Looking further back, for the first time Yılmaz and coworkers showed AMY1 copy expansion in some Neandertal genomes, and one Denisovan. These archaic people and recent hunter-gatherer groups had the potential for greater salivary amylase expression, suggesting they shared some history of selection for increased starch consumption, if less intense than in agricultural groups. The researchers hypothesized a greater availability and value of starch in hominin diets would have coincided with the routine cooking of starchy foods, possibly in the period before one million years ago.
Denisovan genes and brain biology
The phenotypic effects of Neandertal genes have become more and more a subject of research. But similar research on Denisovan genes has been more challenging in large part because living people in island Southeast Asia and Oceania, who have the highest proportion of Denisovan ancestry, are underrepresented in human genetics studies. This year a study by Danat Yermakovich and collaborators added a new perspective by sampling 74 people from Daru Island on the southern Papua coast, and 54 people from Mt. Wilhelm on Papua's highland spine. They applied statistical methods to identify segments introgressed from Denisovan and Neandertal populations, and compared these with broader datasets from Eurasia.
DNA regions with statistical support for introgression from Denisovans and Neandertals added up to around 40 megabases per individual. Twenty-one archaic haplotypes had high frequencies above 70%, and many contain no protein-coding regions. When Yermakovich and coworkers compared highland and lowland samples, they found they differed a bit more from each other in archaic parts of their genomes. For the Daru Island sample, higher-frequency Denisovan haplotypes were associated with immune function. For the highlanders, the researchers noted: “Highly differentiated haplotypes that exhibited higher frequencies in highlanders overlapped several genes associated with early brain development, a result also observed for some of the most highly differentiated nonarchaic haplotypes in these populations.”
Like most studies of possible phenotypic consequences of archaic ancestry, this one hints at some dynamics that will require larger samples to test. The researchers suggest that the early settlement of the highlands may have exerted pressure on early development due to hypoxia, possibly a parallel to the effect of the well-known Denisovan EPAS haplotype in the high-altitude populations of Nepal and Tibet. Both immunity in the lowlands and development in the highlands may be parts of human biology where new selective pressures made use of variation from archaic people.
A story of proto-Indo-European
For the last decade, researchers have been rewriting the story of the origin and initial differentiation of the Bronze Age peoples who may have spoken early Indo-European languages. A wide array of studies have shown a massive influx of steppe ancestry into groups in Europe, across Central Asia, and into the Indian subcontinent. The findings have supported the idea that this ancestry originated from people who produced an archaeological culture known as the Yamnaya, in the steppes north of the Black and Caspian Seas, possibly the proto-Indo-European source.
But some loose ends remained. One unknown was the connection with the Indo-Anatolian languages, including Hittite, which split off earlier than the main Indo-European branches. Another was the formation of the Yamna population itself.
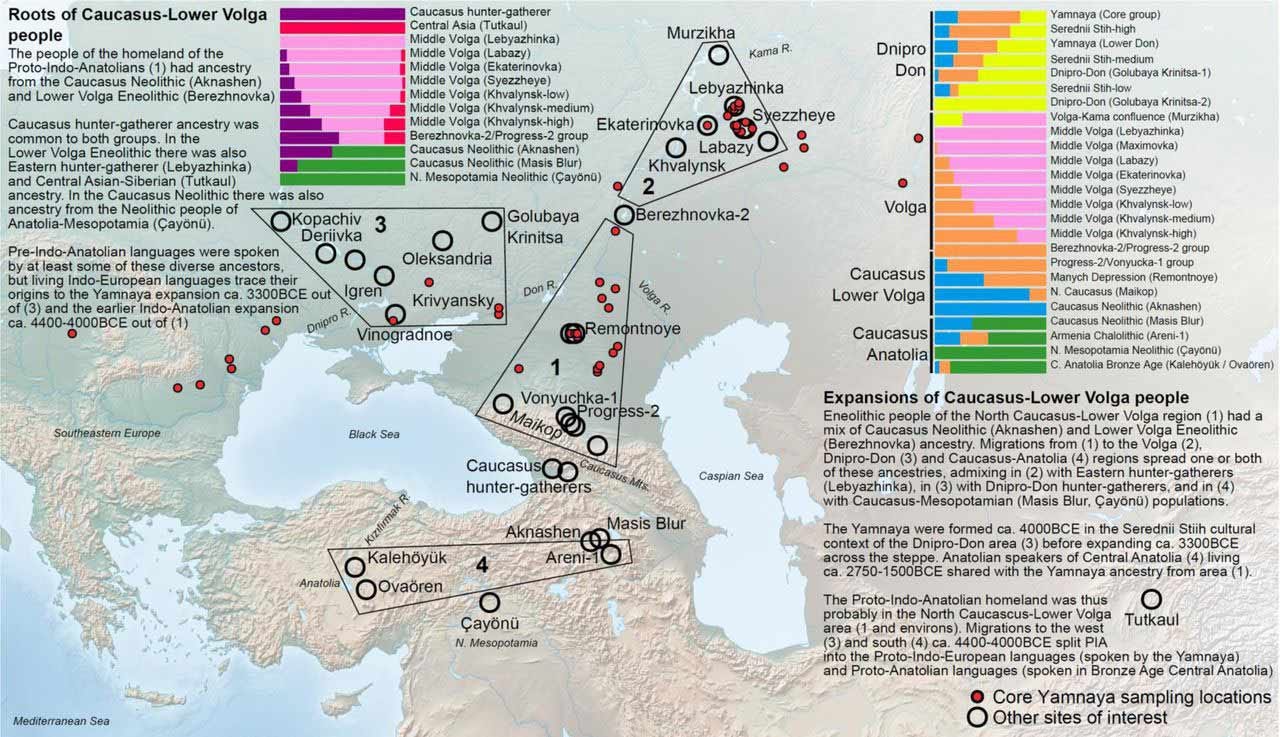
Early this year, two preprints led by Iosif Lazaridis and Alexey Nikitin provided new evidence about the formation and early dispersals of the population that developed Yamnaya culture. The work assessed genomes of ancient people from the North Pontic Region, spanning the areas of modern Ukraine and Russia north of the Black Sea, Caucasus, and Caspian Sea. From Neolithic into Bronze Age times, the teams of researchers document the formation of a cline of variation stretching from the Caucasus northward to the lower Volga River, which they label the CLV cline.
This interconnected region was the source of three pulses of gene flow across the broader Pontic Steppe. Nikitin and coworkers point out that the pattern of three waves of population contacts was predicted by the archaeologist Marija Gimbutas, one of the earliest advocates of the idea of an early Bronze Age origin of the proto-Indo-European language in this region.
Meanwhile, later in the year a preprint led by Fulya Yediay looked at a slightly later branch within the Indo-European family: the division between languages that came to exist in the eastern and western Mediterranean. They found a direct Yamnaya input into the Bronze Age populations of Greece and Armenia, while western groups in Spain, France, and Italy had steppe ancestry that had come to them via the Bell Beaker culture of central and western Europe. These different pathways of ancestry from the Pontic steppe region mark different patterns of connections, possibly giving rise to this early stage of linguistic and cultural differentiation.
Deep history of India from genomes of living people
Ancient DNA has made a big difference to understanding ancient population origins and mixture, but larger samples of whole genomes from living people still have much to offer. This is especially true in regions of the world that have been underrepresented in studies of human genetic variation. Elise Kerdoncuff and coworkers presented a study in February of more than 2700 whole genomes of research participants from India, the largest sample yet assembled from this region. The sample has been gathered for a large-scale study of aging, and this first study describes the population diversity represented in the sample.
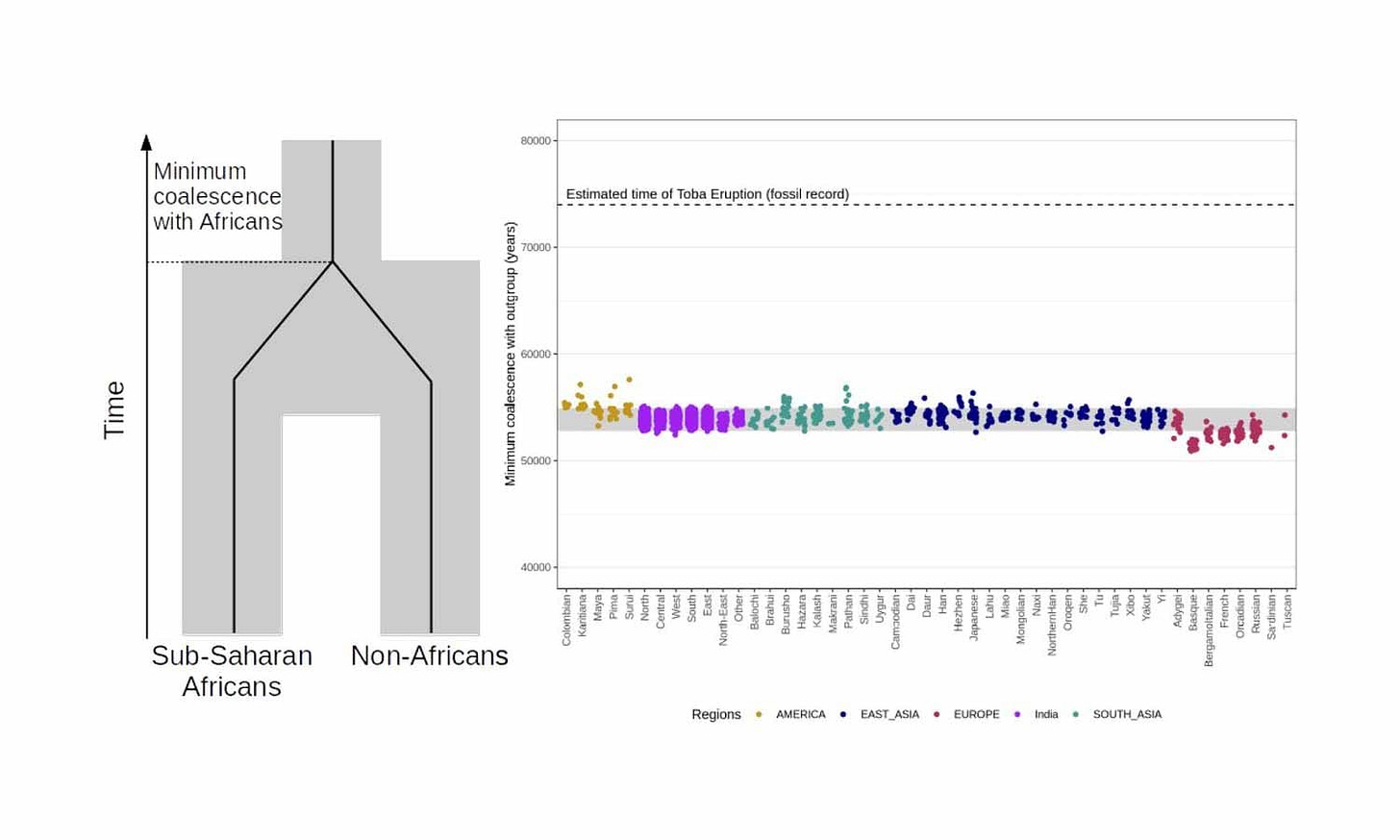
With this large sample of contemporary people, Kerdoncuff and collaborators could try to pin down some of the deep history of the population of the subcontinent. Previous work based on mtDNA had suggested the possibility of very early modern human habitation and population growth in India, well before 60,000 years ago. The new whole-genome data excludes any substantial contribution from populations predating the Toba volcanic eruption 73,000 years ago, and shows that the population of India has the same history of expansion after 53,000 years ago as other Asian populations. In more recent history, the study confirms previous work showing an ancestry cline across northern to southern India, with additional resolution showing mixture with east and southeast Asian populations in northeast India.
For me, a very interesting part of the study was a broader look at Neandertal and Denisovan ancestry in India. Even though the level of Denisovan ancestry in India is no higher than other mainland Asian populations, any large sample can provide new information about Denisovan ancestry. In northeast India, gene flow has connected today's people with populations to the east, yielding a slightly higher Denisovan ancestry. Like those other populations, the Denisovan ancestry comes from two genetically divergent groups, one like the Denisova 3 genome and one quite different. In tune with the findings on early population growth, the study found that the Indian sample had similar Neandertal ancestry as other Eurasian populations. This helps cement the hypothesis that a single pulse of mixture is responsible for most surviving Neandertal DNA today.
One brief Neandertal wave
In December, two studies provided new detail about the mixture of Neandertal genes into today's human groups. One of these, by Leonardo Iasi and coworkers, established a more precise timeline for Neandertal mixture by looking at the decay of Neandertal haplotypes in ancient DNA samples. After the entry of these haplotypes into human groups, recombination makes them shorter and shorter over time. Iasi and collaborators found that today's people everywhere have DNA from the same brief period of mixture with Neandertals, which they place into a 7000-year-long window centered on 47,000 years ago.
The second study by Arev Sümer and coworkers obtained DNA data from 45,000-year-old bones from Ranis, Germany and Zlatý kůň, Czechia. The genomes reveal that these bones represent an early branch from the founder population that gave rise to all living peoples in Eurasia. The Neandertal component of their ancestry is the same as for other groups, with mixture estimated to have happened between 45,400 and 49,400 years ago.
Together these studies provide a strong basis for a model with a founder population from Africa first entering Eurasia slightly before 50,000 years ago, mixing with Neandertals for a few thousand years, and expanding throughout the rest of the world. The eastern branches of this dispersing population then met and mixed with Denisovans. Notably, no genetic data have shown that any of today's groups of Eurasia, island Southeast Asia, or Oceania have substantial input from any earlier migration of modern people from Africa.
Already sparking controversy, these findings seem to conflict with archaeological evidence for human presence in Australia by 65,000 years ago, as well as a broad array of early modern remains from Israel to China dating to earlier than 50,000 years ago. Reconciling these lines of evidence is likely to lead to some new ways of looking at this critical period in our recent evolution.
Our globular brains
Modern people, Neandertals, and other late archaic people have roughly similar brain sizes, but the brains of modern people tend to reflect their more rounded skull, more spherical and compact from front to back. This rounded modern shape has been dubbed globular. Exactly why and how early modern people evolved this way is not clear.
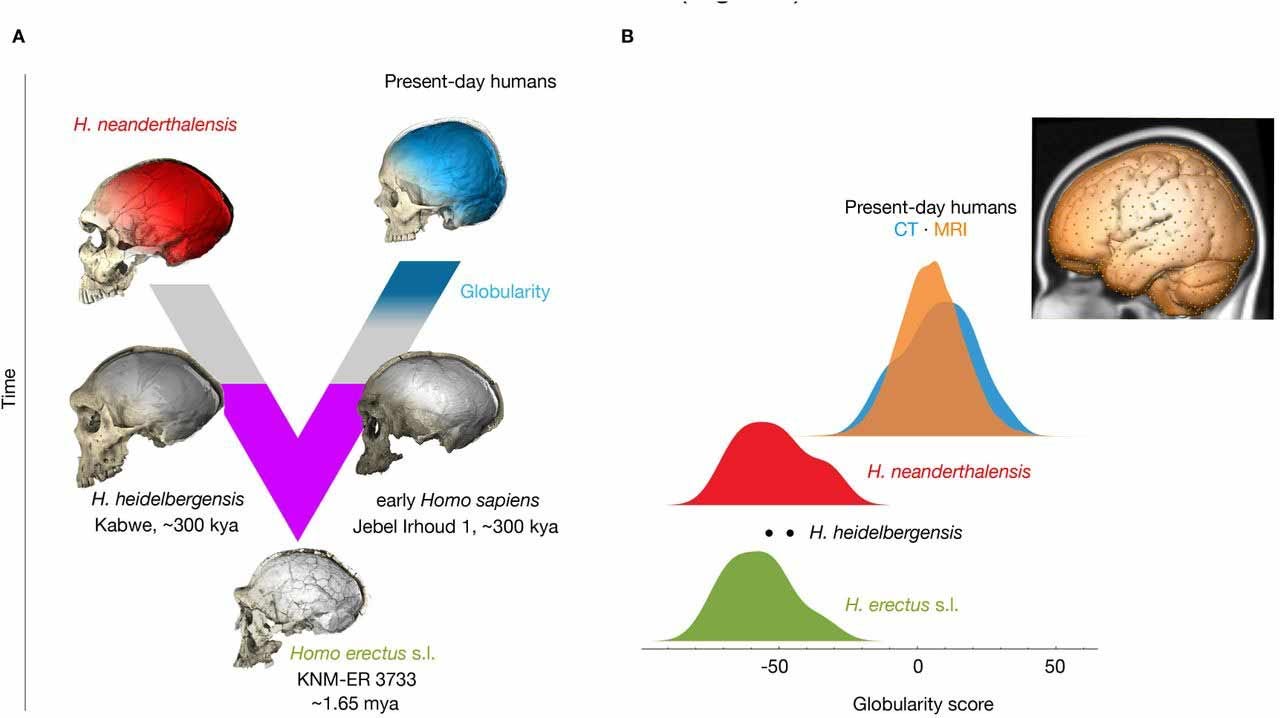
A study this year by Barbara Molz and coworkers incorporated MRI and CT data from more than 30,000 people to understand the genetic component of variation in this globular cranial shape in living people. The study developed a measure of globular shape, and found that this had a similar fraction of additive genetic variation as other, more traditional measures of cranial areas. But unlike earlier work, they found that genes inherited by recent people from Neandertals have no significant relationship with globular shape today. They suggest that despite the very large sample size, the study may still be underpowered to find effects of low-frequency Neanderthal genetic variants.
The study's most interesting finding was that the genetic loci statistically associated with globularity include several connected with the brain's ventricular system, and expressed early in fetal and childhood development. Molz and collaborators suggest that the operation of hydrostatic pressure early in brain development might have been the mechanism for evolution of the modern, globular cranial shape.
Time of the chumanobos
Human ancestors diverged from the common ancestors of chimpanzees and bonobos around six million years ago, more or less. Anthropologists have never yet found any fossil representatives of this stem population. Or so we think. That conclusion depends a bit on exactly how, when, and where these ancestors actually split.
Nick Patterson and coworkers in 2006 suggested that the human and chimpanzee-bonobo lineages had a long, drawn-out divergence with hybridization mixing the human and chimpanzee-bonobo lineages for more than a million years. That idea has been controversial over the years. But it finds new support this year in a study from Trevor Cousins and coworkers. Using genome sequences from humans, chimpanzees, and bonobos, they noted that all three species share a pattern that looks like a big bubble of population size between around 5 million and 7 million years ago. The same pattern is not present in gorilla genomes, nor in either Sumatran nor Bornean orangutans, suggesting a real change leading up to the human-chimpanzee-bonobo divergence.
It's possible that the human-chimpanzee-bonobo common ancestors were just a really big population—ten times the size of any of these species for most of their history. But another solution is that the population was highly structured: It consisted of divergent smaller populations that were partially isolated with limited gene flow for a very long time.
This is another of the areas of developing research I'm happy to see. In one of my first scientific projects I worked to understand the population size of this human-chimpanzee-bonobo ancestral stem. I've contributed to similar research since then, helping to demonstrate the extensive incomplete lineage sorting in great ape speciation. With the emerging understanding that remixing of divergent population branches has been common in our evolution, this work may help us see fossils like Sahelanthropus and Ardipithecus in a new light.
Deeper reading
Several other areas of interesting research progressed this year, and I've read a lot of interesting studies that I might have included. They'll likely continue to develop in 2025.
Work on ancient pathogens, which I covered last year, is growing by leaps and bounds. I was especially fascinated this year by developing work on circulating viruses that have recently invaded human genomes, just starting to become visible in ancient remains. This year's studies on the dispersal of treponemal diseases have also generated some provocative findings, that I'll write about in an upcoming post.
As I wrote last year, the development of community involvement and leadership in ancient DNA research remains of key importance to the future of the field. At the end of 2024, a new article by Anna Källén and collaborators highlights some of the ways that practices in ancient DNA research sometimes falls short of best practices for heritage research. The ideal of collaborative interdisciplinary research across genetics, archaeology, and history is challenging in practice to create and maintain, especially with a funding structure that favors large centralized research institutions. Some of the most exciting new research is building leadership within the nations and communities where heritage resides. More of this research in 2025 will keep moving the understanding of past populations forward.
References
Akbari, A., Barton, A. R., Gazal, S., Li, Z., Kariminejad, M., Perry, A., Zeng, Y., Mittnik, A., Patterson, N., Mah, M., Zhou, X., Price, A. L., Lander, E. S., Pinhasi, R., Rohland, N., Mallick, S., & Reich, D. (2024). Pervasive findings of directional selection realize the promise of ancient DNA to elucidate human adaptation. bioRxiv, 2024.09.14.613021. https://doi.org/10.1101/2024.09.14.613021
Baker, B. J., Crane-Kramer, G., Dee, M. W., Gregoricka, L. A., Henneberg, M., Lee, C., Lukehart, S. A., Mabey, D. C., Roberts, C. A., Stodder, A. L. W., Stone, A. C., & Winingear, S. (2020). Advancing the understanding of treponemal disease in the past and present. American Journal of Physical Anthropology, 171(S70), 5–41. https://doi.org/10.1002/ajpa.23988
Cousins, T., Schweiger, R., & Durbin, R. (2024). Deep coalescent history of the hominin lineage (p. 2024.10.17.618932). bioRxiv. https://doi.org/10.1101/2024.10.17.618932
Fortier, A. L., & Pritchard, J. K. (2024a). Ancient trans-species polymorphism at the Major Histocompatibility Complex in primates (p. 2022.06.28.497781). bioRxiv. https://doi.org/10.1101/2022.06.28.497781
Fortier, A. L., & Pritchard, J. K. (2024b). The primate Major Histocompatibility Complex: An illustrative example of gene family evolution (p. 2024.09.16.613318). bioRxiv. https://doi.org/10.1101/2024.09.16.613318
Iasi, L. N. M., Chintalapati, M., Skov, L., Mesa, A. B., Hajdinjak, M., Peter, B. M., & Moorjani, P. (2024). Neandertal ancestry through time: Insights from genomes of ancient and present-day humans (p. 2024.05.13.593955). bioRxiv. https://doi.org/10.1101/2024.05.13.593955
Irving-Pease, E. K., Refoyo-Martínez, A., Barrie, W., Ingason, A., Pearson, A., Fischer, A., Sjögren, K.-G., Halgren, A. S., Macleod, R., Demeter, F., Henriksen, R. A., Vimala, T., McColl, H., Vaughn, A. H., Speidel, L., Stern, A. J., Scorrano, G., Ramsøe, A., Schork, A. J., … Willerslev, E. (2024). The selection landscape and genetic legacy of ancient Eurasians. Nature, 625(7994), 312–320. https://doi.org/10.1038/s41586-023-06705-1
Källén, A., Mulcare, C., Nyblom, A., & Strand, D. (2024). Petrous Fever. Current Anthropology, 000–000. https://doi.org/10.1086/733328
Kerdoncuff, E., Skov, L., Patterson, N., Zhao, W., Lueng, Y. Y., Schellenberg, G. D., Smith, J. A., Dey, S., Ganna, A., Dey, A. B., Kardia, S. L. R., Lee, J., & Moorjani, P. (2024). 50,000 years of Evolutionary History of India: Insights from ∼2,700 Whole Genome Sequences (p. 2024.02.15.580575). bioRxiv. https://doi.org/10.1101/2024.02.15.580575
Lazaridis, I., Patterson, N., Anthony, D., Vyazov, L., Fournier, R., Ringbauer, H., Olalde, I., Khokhlov, A. A., Kitov, E. P., Shishlina, N. I., Ailincăi, S. C., Agapov, D. S., Agapov, S. A., Batieva, E., Bauyrzhan, B., Bereczki, Z., Buzhilova, A., Changmai, P., Chizhevsky, A. A., … Reich, D. (2024). The Genetic Origin of the Indo-Europeans (p. 2024.04.17.589597). bioRxiv. https://doi.org/10.1101/2024.04.17.589597
Molz, B., Eising, E., Alagöz, G., Schijven, D., Francks, C., Gunz, P., & Fisher, S. E. (2024). Imaging genomics reveals genetic architecture of the globular human braincase (p. 2024.03.20.585712). bioRxiv. https://doi.org/10.1101/2024.03.20.585712
Moreno-Mayar, J. V., Sousa da Mota, B., Higham, T., Klemm, S., Gorman Edmunds, M., Stenderup, J., Iraeta-Orbegozo, M., Laborde, V., Heyer, E., Torres Hochstetter, F., Friess, M., Allentoft, M. E., Schroeder, H., Delaneau, O., & Malaspinas, A.-S. (2024). Ancient Rapanui genomes reveal resilience and pre-European contact with the Americas. Nature, 633(8029), 389–397. https://doi.org/10.1038/s41586-024-07881-4
Nikitin, A. G., Lazaridis, I., Patterson, N., Ivanova, S., Videiko, M., Dergachev, V., Kotova, N., Lillie, M., Potekhina, I., Krenz-Niedbała, M., Łukasik, S., Makhortykh, S., Renson, V., Shephard, H., Sirbu, G., Svyryd, S., Tkachuk, T., Włodarczak, P., Callan, K., … Reich, D. (2024). A genomic history of the North Pontic Region from the Neolithic to the Bronze Age (p. 2024.04.17.589600). bioRxiv. https://doi.org/10.1101/2024.04.17.589600
Patterson, N., Richter, D. J., Gnerre, S., Lander, E. S., & Reich, D. (2006). Genetic evidence for complex speciation of humans and chimpanzees. Nature, 441(7097), 1103–1108. https://doi.org/10.1038/nature04789
Rothschild, B. M., Hershkovitz, I., & Rothschild, C. (1995). Origin of yaws in the Pleistocene. Nature, 378(6555), 343–344. https://doi.org/10.1038/378343b0
Schiffels, S., & Nägele, K. (2024). Rapa Nui’s population history rewritten using ancient DNA. Nature, 633(8029), 290–291. https://doi.org/10.1038/d41586-024-02620-1
Sümer, A. P., Rougier, H., Villalba-Mouco, V., Huang, Y., Iasi, L. N. M., Essel, E., Mesa, A. B., Furtwaengler, A., Peyrégne, S., de Filippo, C., Rohrlach, A. B., Pierini, F., Mafessoni, F., Fewlass, H., Zavala, E. I., Mylopotamitaki, D., Bianco, R. A., Schmidt, A., Zorn, J., … Krause, J. (2024). Earliest modern human genomes constrain timing of Neanderthal admixture. Nature, 1–3. https://doi.org/10.1038/s41586-024-08420-x
Yediay, F. E., Kroonen, G., Sabatini, S., Frei, K. M., Frank, A. B., Pinotti, T., Wigman, A., Thorsø, R., Vimala, T., McColl, H., Moutafi, I., Altinkaya, I., Ramsøe, A., Gaunitz, C., Renaud, G., Martin, A. M., Demeter, F., Scorrano, G., Canci, A., … Willerslev, E. (2024). Ancient genomics support deep divergence between Eastern and Western Mediterranean Indo-European languages (p. 2024.12.02.626332). bioRxiv. https://doi.org/10.1101/2024.12.02.626332
Yermakovich, D., André, M., Brucato, N., Kariwiga, J., Leavesley, M., Pankratov, V., Mondal, M., Ricaut, F.-X., & Dannemann, M. (2024). Denisovan admixture facilitated environmental adaptation in Papua New Guinean populations. Proceedings of the National Academy of Sciences, 121(26), e2405889121. https://doi.org/10.1073/pnas.2405889121
Yilmaz, F., Karageorgiou, C., Kim, K., Pajic, P., Scheer, K., Human Genome Structural Consortium, Beck, C. R., Torregrossa, A.-M., Lee, C., & Gokcumen, O. (2024). Reconstruction of the human amylase locus reveals ancient duplications seeding modern-day variation. Science, 386(6724), eadn0609. https://doi.org/10.1126/science.adn0609