New insights into the biology of Homo luzonensis
Studies of teeth from Callao Cave yield information about the pace of development in this species and its possible connections with Homo erectus.
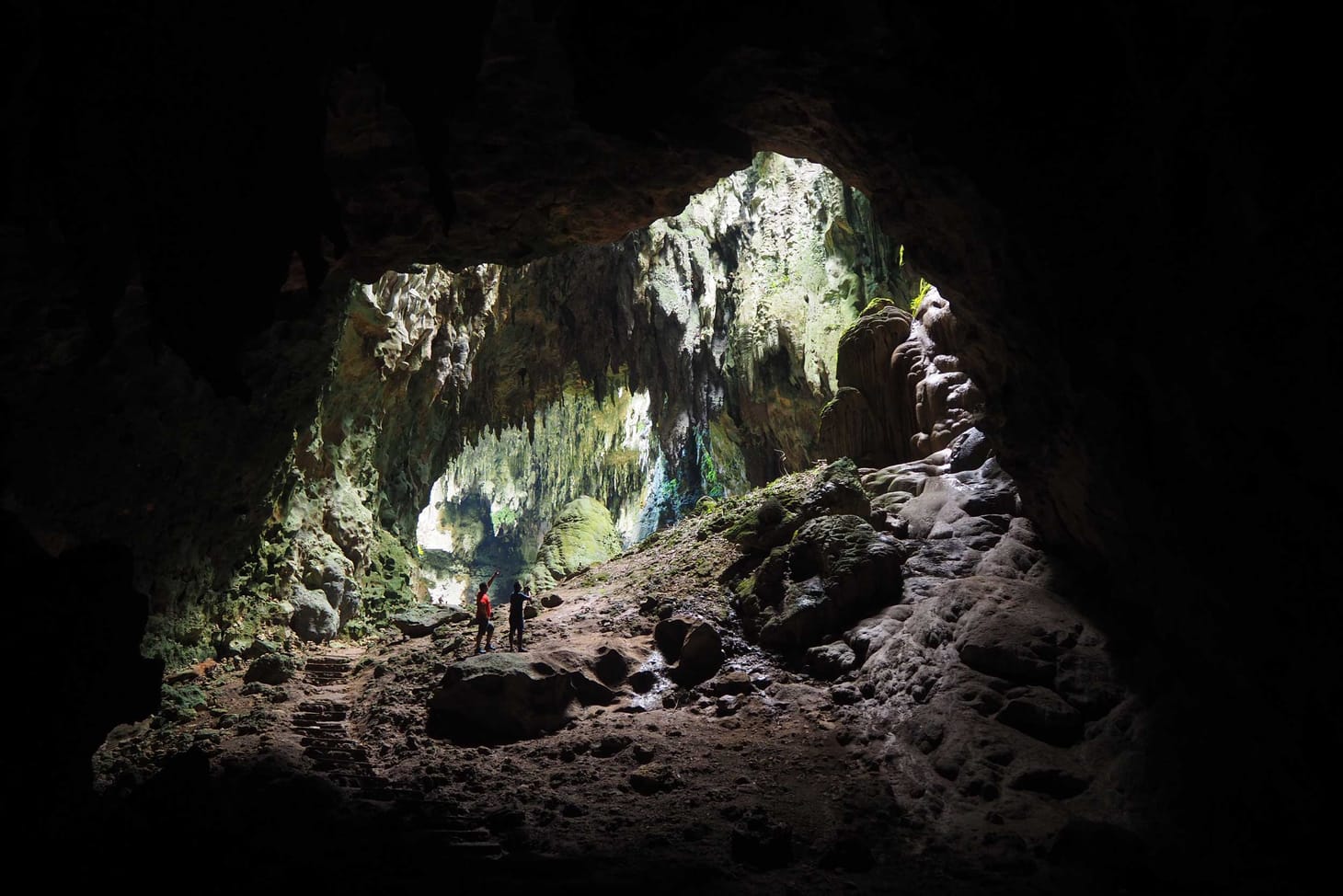
In 2019 Florent Détroit and coworkers described fossil hominin teeth and some postcranial fossils from Callao Cave, on the island of Luzon in the Philippines and dating to around 67,000 years old. The first piece was a single foot bone found in a decade earlier in excavations led by the archaeologist Armand Mijares. The piece gave little to go on, other than its very small size. Mijares continued working and building more and more information about the site, ultimately finding teeth that are different from recent humans, Homo erectus and other hominin species. The team considered the fossils to represent a previously-unknown species, Homo luzonensis.
This was the second extinct human relative discovered from the islands of Wallacea. The first was Homo floresiensis, discovered in Liang Bua on the island of Flores, Indonesia. Most of the fossils attributed to H. floresiensis are between around 100,000 and 60,000 years old, a range that overlaps with the Callao Cave fossils of H. luzonensis. Both samples represent small-bodied hominins.
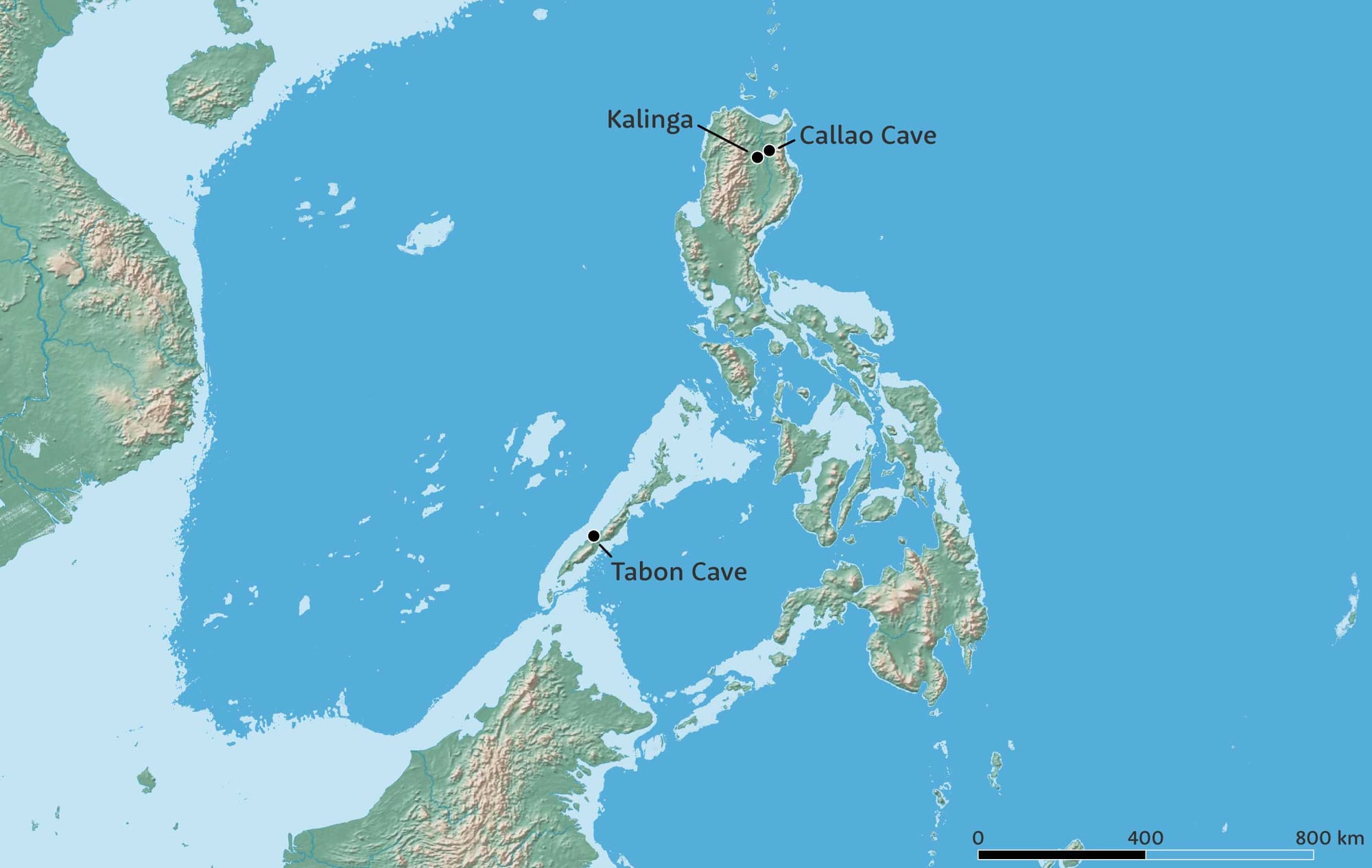
Did these fossil groups both evolve from a shared ancestor, such as Homo erectus? It is hard to answer this question because both Flores and Luzon were inhabited for a very long time. Sites on Flores preserve stone artifacts as old as a million years. Stone tools and a butchered rhinoceros from Kalinga, Luzon, show that hominins were on this island by around 700,000 years ago. We don't know yet whether the fossils from 65,000 years ago evolved from the earliest inhabitants of these islands. But if they did, those populations had a very long time to evolve idiosyncratic features that might obscure their relationship.
Recently, Anneke van Heteren and coworkers released a preprint reporting on the pace of development and age of one of the Callao Cave individuals, the individual numbered CCH6. They put teeth into a synchrotron beamline to obtain high-resolution images of the cementum around their roots. This new work prompted me to look back at another recent paper, in which Clément Zanolli and coworkers compared the internal details of the Callao Cave teeth with Homo floresiensis and other species. That study gives some insight into the possible ancestry of the two groups.
An accelerated pace of dental eruption
Cementum is a mineralized tissue that forms on the roots of teeth, where it provides an attachment between the root and the surrounding alveolus, or socket, within the bone of the maxilla or mandible. Cementum is alive. Its cells, called cementocytes, grow in a similar way to the osteoblasts that form bone. Cementum grows slowly after a tooth erupts and forms extremely thin annual layers, which are called lines of Salter. Counting these layers on a tooth root provides an estimate of the number of years between the eruption of the tooth and the individual's death. Added to information about the age at tooth eruption, this becomes an age-at-death estimator.
Last year I wrote about the analysis of cementum from Neanderthal teeth from Krapina, Croatia, where Paola Cerrito and collaborators examined the growth of cementum using synchrotron scanning. That study confirmed the overall young age at death for the Krapina population. It also gave insight into the timing of some significant stress events in the lifetimes of individuals, which may have corresponded to menarche or birth of a child in women's lives.
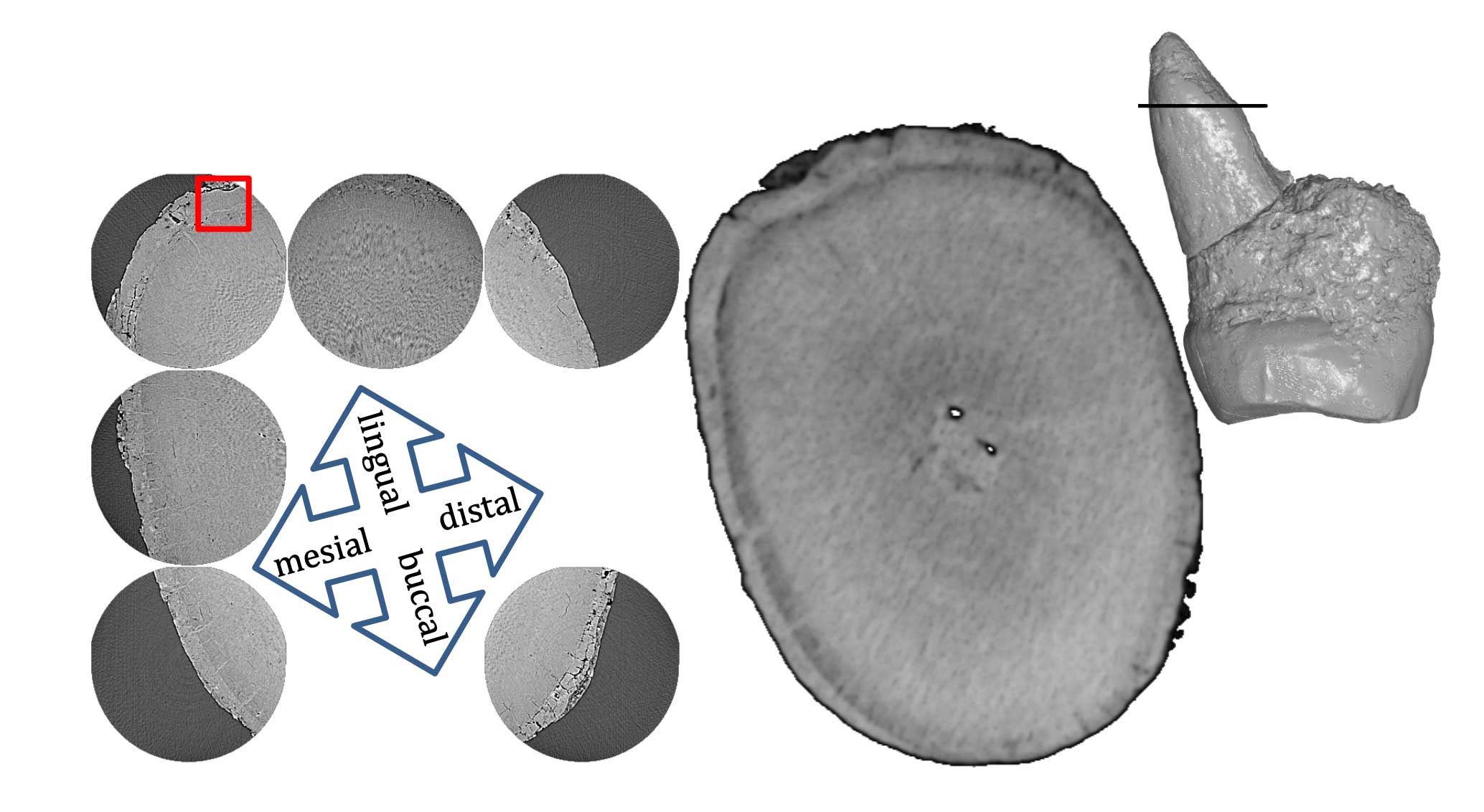
In the study by van Heteren and coworkers, they focused on the differences in the number of cementum increments in the roots of different teeth. The teeth all come from one individual, and so the differences in cementum layers between two teeth reflect the number of years between the eruption of the teeth. The first permanent molar erupts first. In humans today this is followed by the premolars and second molar. Chimpanzees erupt their teeth at younger ages, and the premolars are generally later than the second molar. The years in this sequence and order of premolar and second molar eruption tell something about the pace of development.
“[A] reasonable estimate for the eruption ages of M1, M2 and P4 H. luzonensis would be three, six and seven years old respectively, based on a chimpanzee-like dental development pattern.”—Anneke van Heteren and coworkers
The researchers found that the H. luzonensis first molar eruption preceded the second molar by four years, followed by the fourth premolar a year later. In their assessment, the development of the teeth in this individual was similar to chimpanzees in sequence and pace. Cementum data is not very widely available yet for other hominins besides Neanderthals, so the results cannot be compared directly with H. floresiensis, H. erectus, or other species. But we do know something about the pace of dental development in these species from studies of enamel growth. For example, Tanya Smith and coworkers in a 2015 paper examined the enamel growth of early hominins, showing that first molar eruption in Australopithecus was at approximately the same range of ages as chimpanzees, while Paranthropus first molar eruption averaged slightly later. Christopher Dean and Holly Smith reviewed data for H. erectus dental eruption, suggesting ages around 4 years for first molar eruption and 8 years for second molar eruption.
From this comparative perspective, the dental development of the CCH6 individual of H. luzonensis was not very different from Australopithecus or Homo erectus. One explanation proposed for the smaller body sizes of H. luzonensis and H. floresiensis is that smaller size enabled faster development. That is, individuals that matured earlier could start reproducing earlier, and in a growing population natural selection would favor this faster developmental timing. But the cementum data suggest that there may have been little difference in developmental timing between H. erectus and H. luzonensis, meaning that life history may be an unlikely cause of selection for smaller body size.
Still, the cementum data reflect the growth of this tissue after tooth eruption, not how long the teeth took to develop. Possibly future study of the enamel growth increments will give a more complete picture of the development of the first molar, which could provide a timeline back to the birth of the individual.
Probing a link to Homo erectus
The research from Zanolli and coworkers in 2022 examined another aspect of the CCH6 teeth: the enamel-dentin junction. When a tooth forms, the enamel that eventually makes up the crown grows outward incrementally, while dentin forms by mineralization of pre-dentin. The enamel-dentin junction is the interface between these two processes. The different density of enamel and dentin make this boundary visible on microCT images of a tooth.
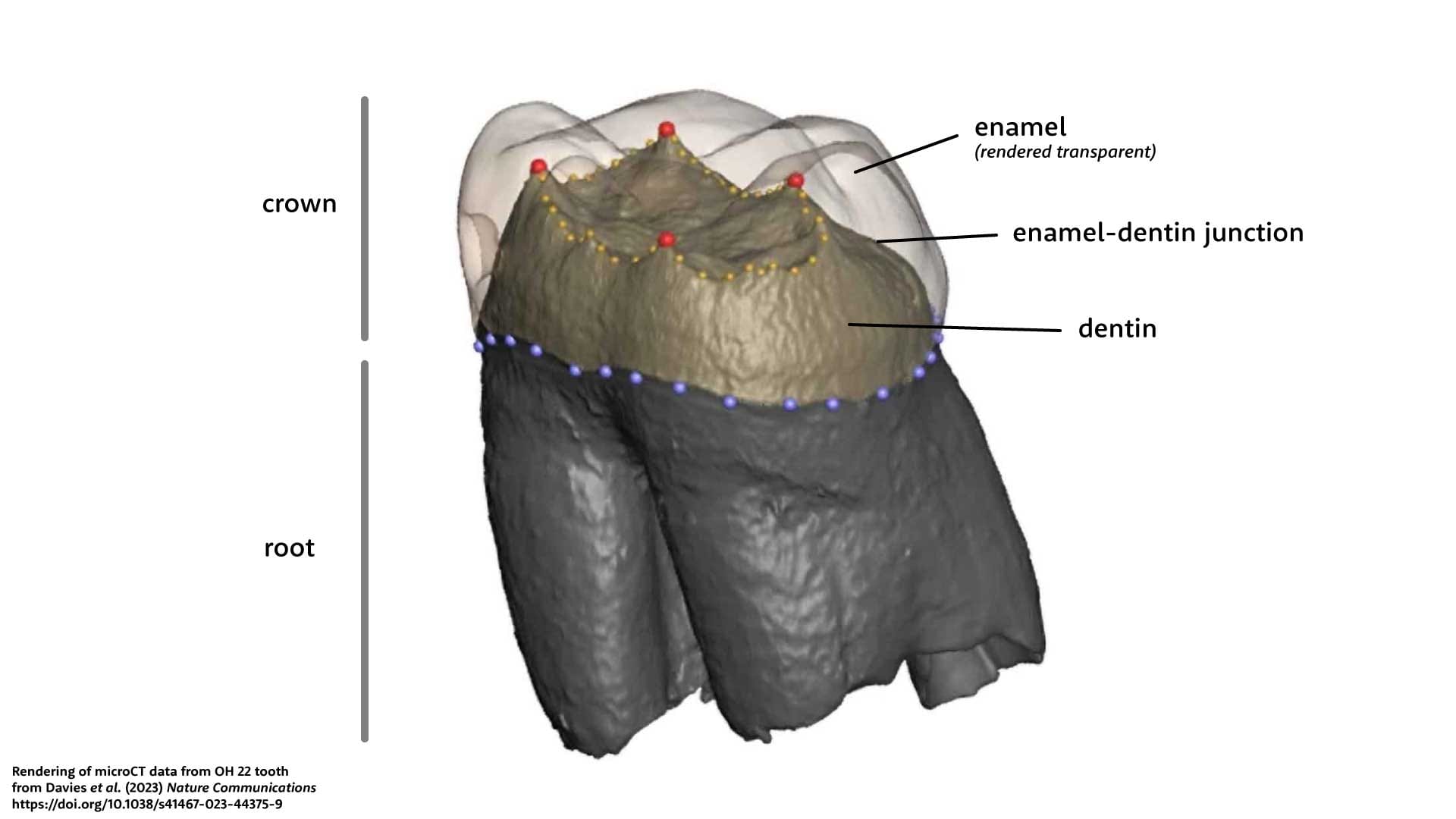
The form of the enamel-dentin junction has its own pattern of variation somewhat correlated with the shape of the overlying crown surface. Normal chewing exposes the tooth crown to wear and other kinds of damage from the time the tooth erupts, changing its visible appearance and making it hard to compare the crown shapes of different individuals. But the enamel-dentin junction is protected inside the tooth until the wear on the tooth's surface becomes fairly extensive, which has made this part of the tooth a valuable research subject for scientists trying to understand hominin relationships.
Only the maxillary teeth of CCH6 and one additional maxillary premolar, CCH8, have been attributed to H. luzonensis. The teeth of CCH6 have an interesting shape and size relationship to other hominins. The molars of this individual are small: smaller than the LB1 individual of Homo floresiensis and smaller on average than the molars of recent people. The CCH6 and CCH8 premolars are a bit larger than these other groups. They manifest a broad “shoulder” on the buccal and lingual sides which resemble the much larger premolars of H. erectus and Neanderthals more than the small premolars of recent people.
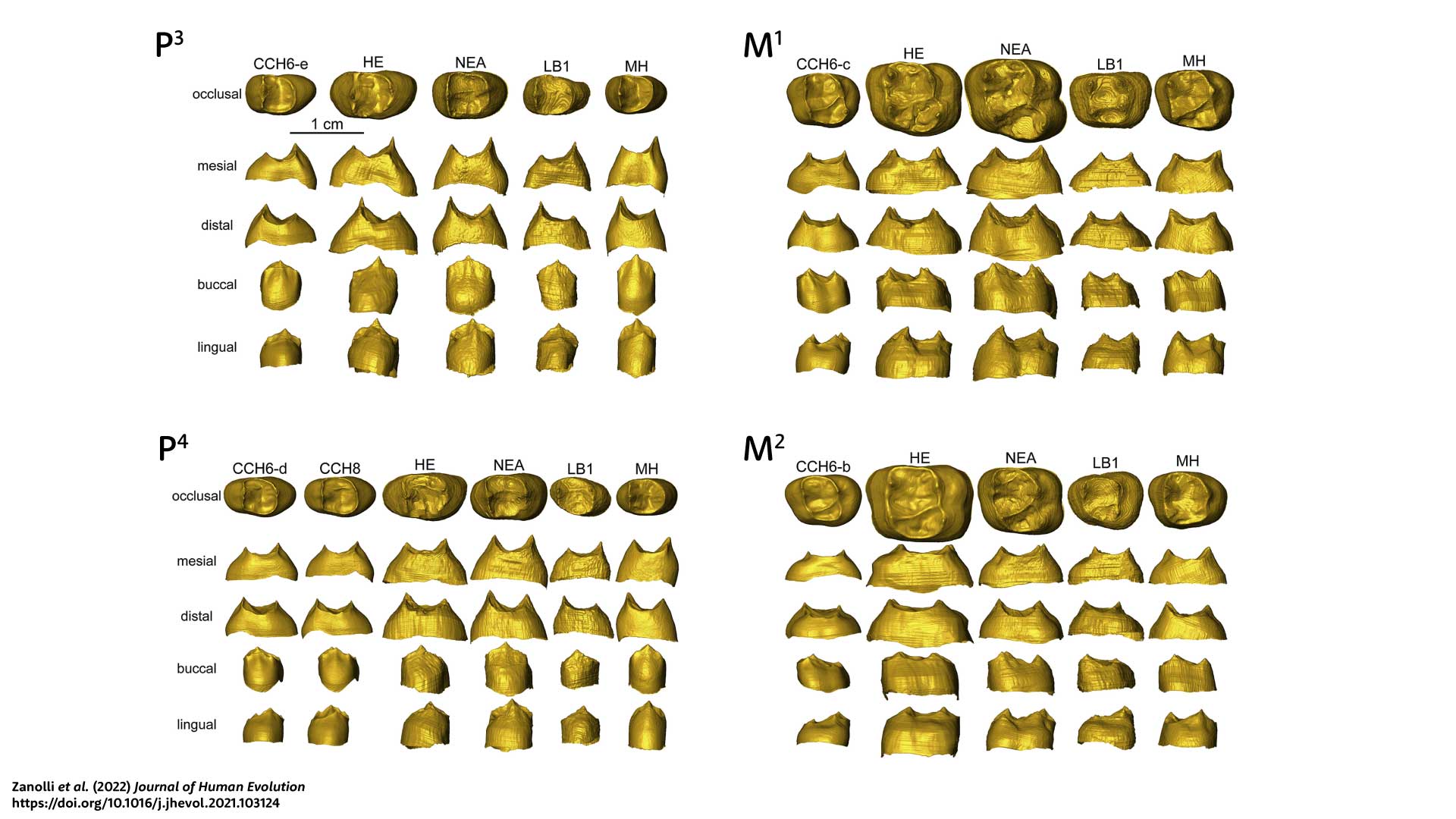
The surface of the enamel-dentin junction has a complex shape and generally scientists try to reduce the dimensions of variation with multivariate statistical methods. In this study, Zanolli and coworkers presented both between-group principal components (bgPCA) and canonical variates (CVA) approaches. Each method produced results for each tooth, and that makes a lot of information to summarize.
For the P3, P4, and M1, the H. luzonensis teeth plot either within the shape range of H. erectus or close to that range. The small size of the CCH6 teeth may be similar or smaller than recent humans, but the shapes of these teeth are not humanlike. The LB1 teeth are not always close to H. luzonensis in these comparisons, although they too tend to be closer to H. erectus than to other groups.
The second molars present a different picture from the other teeth. Both LB1 of H. floresiensis and CCH6 of H. luzonensis share a more similar M2 shape with recent humans than with Neanderthal or H. erectus samples. Like recent humans, both the small-bodied fossil species have second molars smaller than the first molars, and this pattern is different from H. erectus and many Neanderthals.
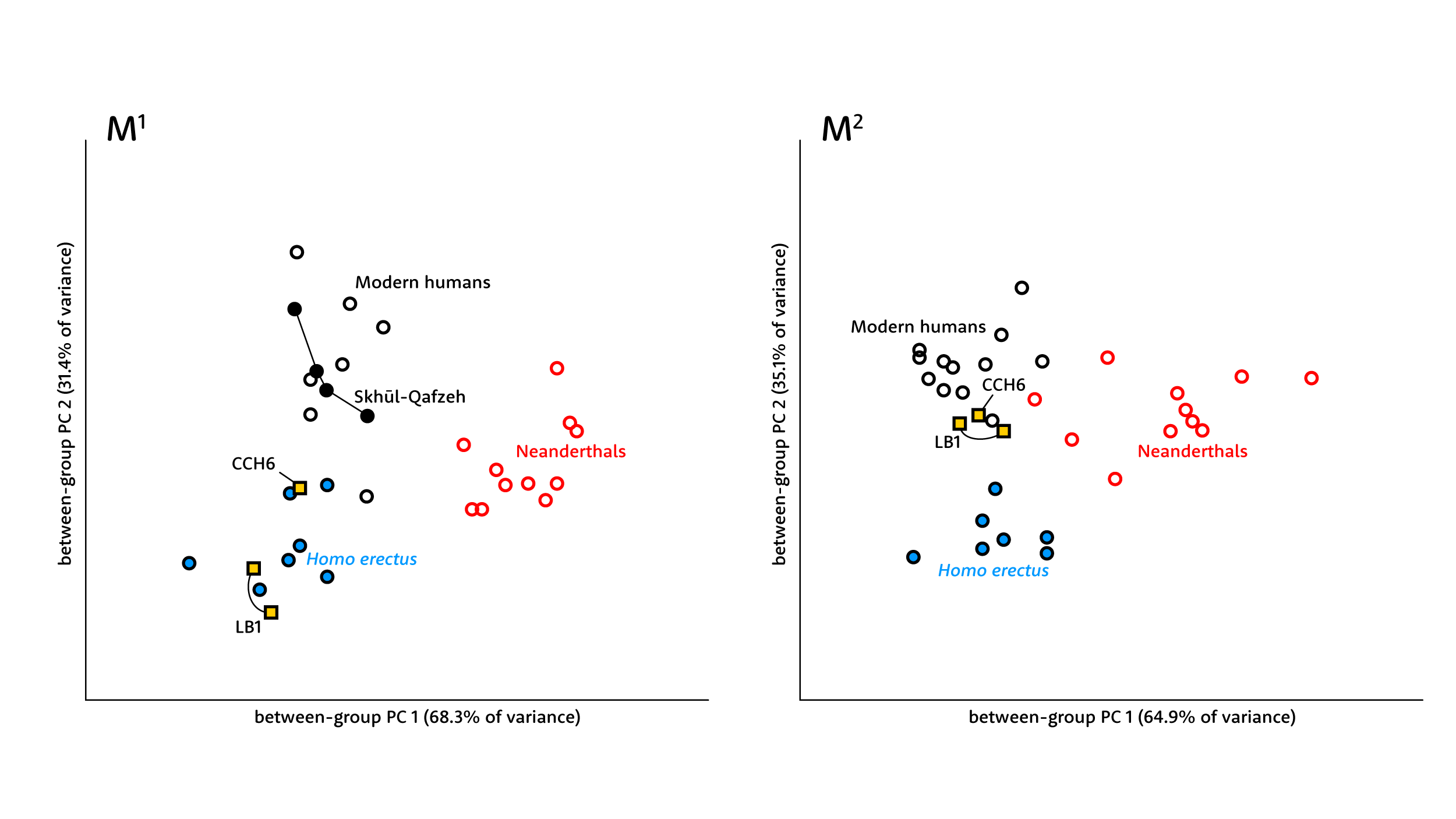
A big omission from these comparisons is the absence of Early Pleistocene species like Homo habilis or Homo rudolfensis. The absence is notable because some studies of other parts of the skeleton have suggested that H. floresiensis may actually have been closer to those very early Homo species than to H. erectus. An alternative explanation for what may seem like shared traits in H. habilis or even Australopithecus is that all of them have small body size, and other traits are correlated with small size. No real phylogenetic comparisons of H. luzonensis have yet been carried out, but the Callao Cave fossils suggest it also had a small body size. So understanding the relationships of H. habilis and other species of Homo with small body sizes is increasingly essential.
To address the lack of enamel-dentin junction information for maxillary teeth of H. habilis, the researchers carried out an analysis of the external outline of the tooth crowns, which they could study without microCT data. These results are fairly unambiguous, showing that most Callao Cave teeth are more similar in outline to H. erectus teeth than to H. habilis teeth. But “more similar” doesn't mean “the same”: H. luzonensis teeth are outside the range of known H. erectus outlines for most teeth. My guess is that outline shapes are correlated with tooth size to some extent. The Callao Cave teeth are smaller than either H. erectus or H. habilis teeth, giving them outlines different to some degree from both.
This kind of analysis is just a first step toward building the data to test the relationships of these species to each other. To examine phylogeny, we need to understand whether similarities in shape are derived, and that requires comparing them to outgroups such as Australopithecus and ideally better data from H. habilis and other species across Homo. The H. erectus sample includes only individuals from Java and China, which are relevant but limited in comparison to the broader picture across the genus. Representation of recent humans within these datasets is likewise meager. Today's humans in island southeast Asia and adjoining regions have a lot of variation in tooth shape, and it will be valuable to see how enamel-dentin junction traits may correlate within more representative samples of variation.
Still, the data have progressed markedly from the time when the linear dimensions of tooth crowns were all the evidence available for most fossil teeth. Enamel-dentin junction shape reflects a developmental process early in life that has evolved somewhat differently among the varied branches of hominins. The data suggest that these processes worked in a similar way in early Homo erectus and in H. luzonensis and H. floresiensis, at least for the premolars and first molars.
Bottom line
Both studies are great illustrations of the ways that today's researchers are pulling more and more information out of fossils. Being able to identify differences between populations from the subtle differences between teeth has opened many questions about the relationships of Pleistocene groups. A faster pace of dental growth and development may suggest that populations like H. luzonensis and H. erectus arrived at slightly different life history solutions from those in slower-developing groups like recent humans and Homo naledi.
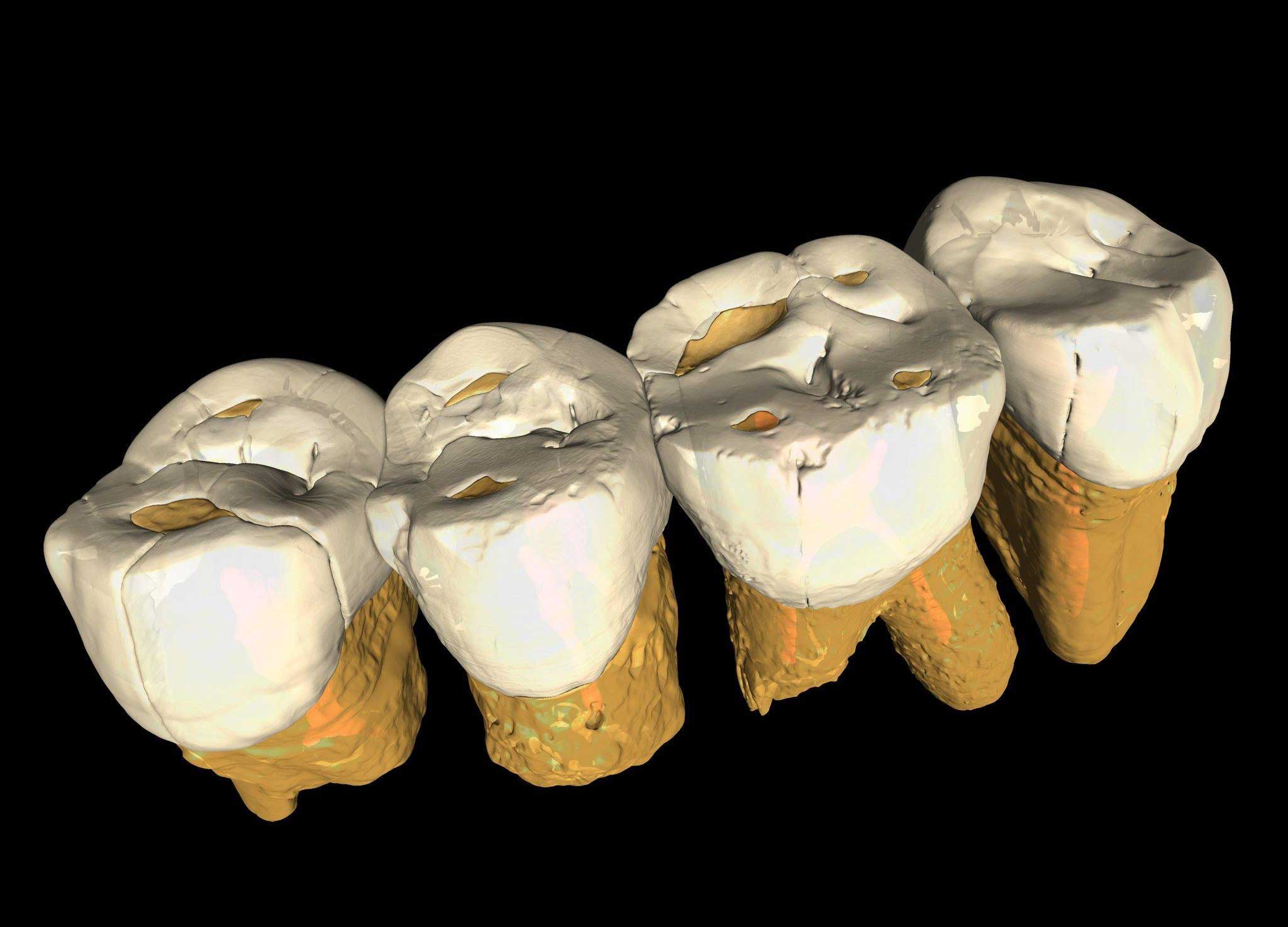
Hominins within and outside of Africa diversified into many populations after the origin of Homo in the earliest Pleistocene. We want to know how their morphology, development, and genetics may have adapted them to their environments, and that's because every one of these populations may help us understand how we evolved ourselves. The first step is understanding the pattern and timeline of branching of these populations and any subsequent interactions.
We don't yet know what part Homo luzonensis played in the broader story. Maybe the population was isolated on Luzon for 700,000 years or longer. Or maybe the hominins of this region continued to interact with each other across some of the narrow straits that separated islands from each other. One thing is for sure: The islands of southeast Asia and Wallacea provide a remarkable set of natural experiments to understand the dispersal, persistence, and local adaptations of hominins.
Notes: The most common question I hear about any ancient hominins is whether there will be DNA evidence anytime soon. This part of the world is one of the more challenging environments for DNA survival in ancient bones due to the warm ambient temperature and high rainfall. Still, DNA has been recovered from a number of Holocene skeletal remains from Wallacea. With advances in technology or broader sampling of sediments, possibly Late Pleistocene sites are not out of reach. Still, even more valuable is broadening the search for more archaeological sites on all these islands.
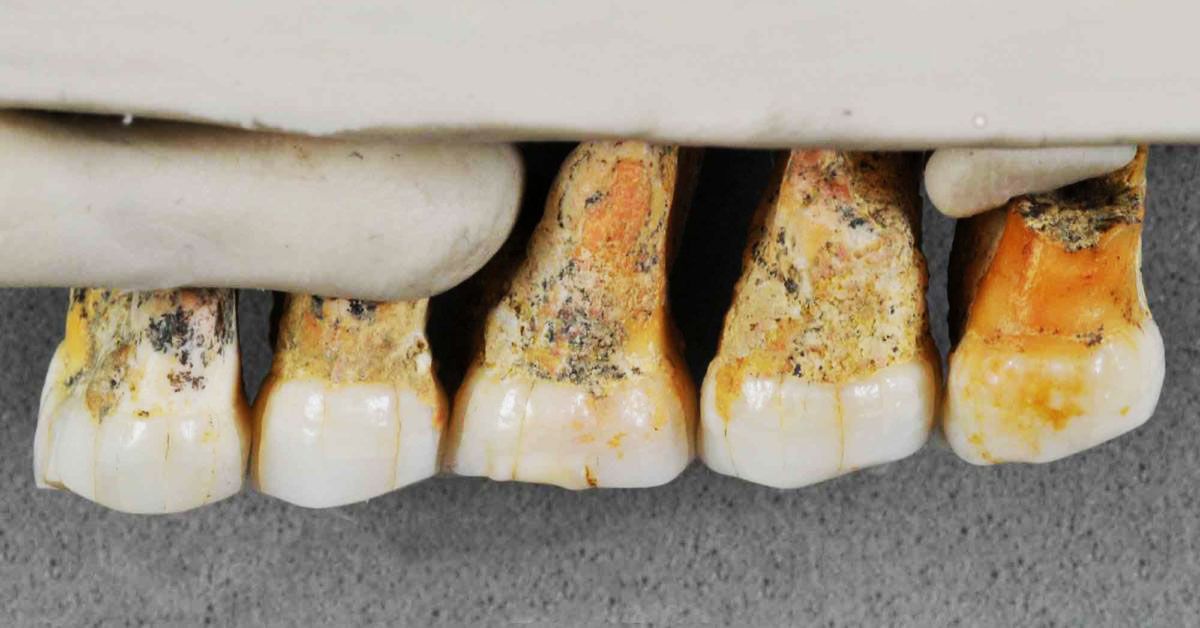
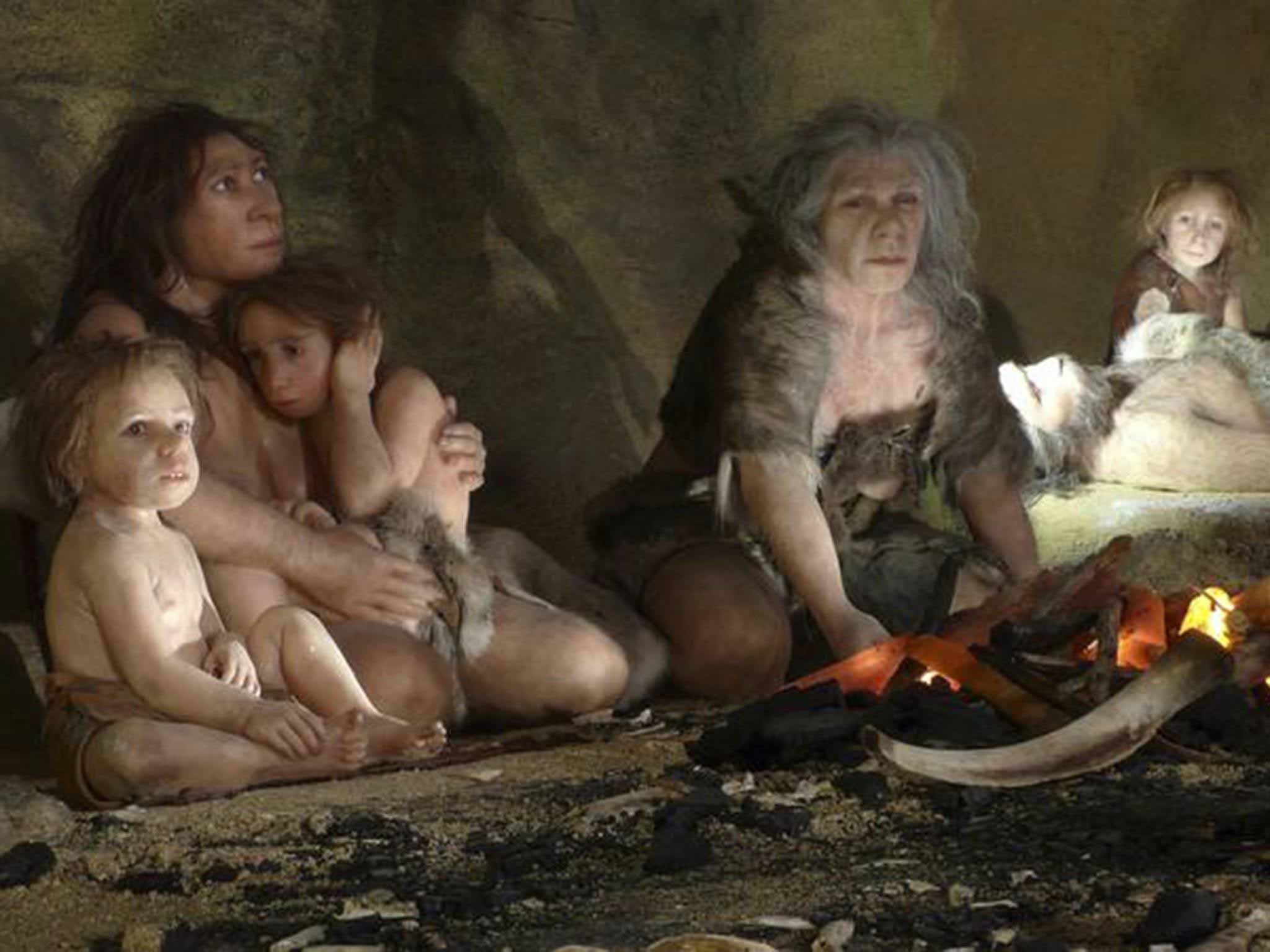
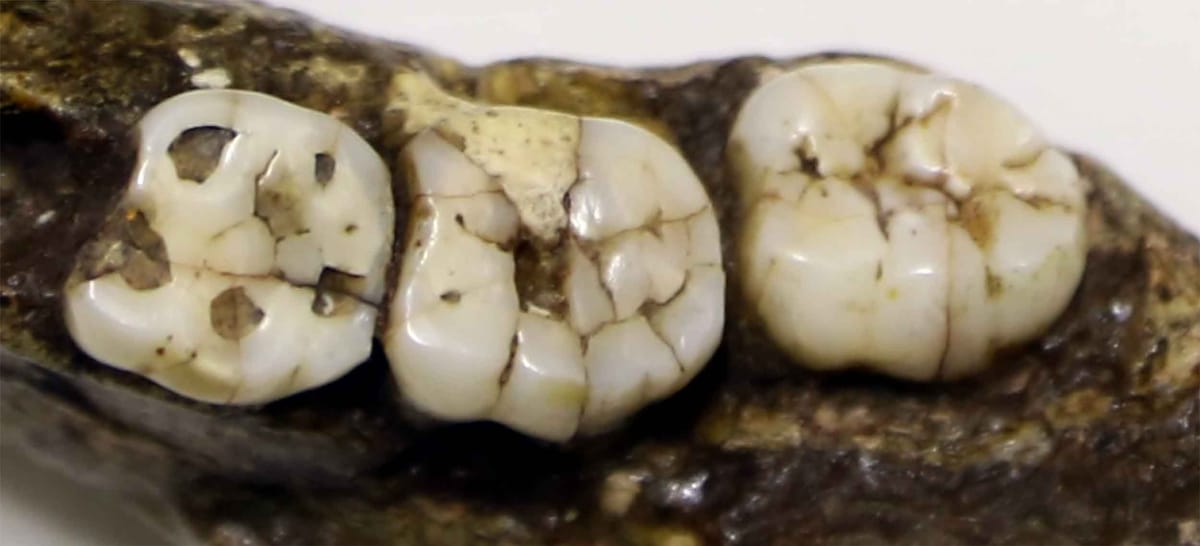
References
Brumm, A., Oktaviana, A. A., Burhan, B., Hakim, B., Lebe, R., Zhao, J., Sulistyarto, P. H., Ririmasse, M., Adhityatama, S., Sumantri, I., & Aubert, M. (2021). Oldest cave art found in Sulawesi. Science Advances, 7(3), eabd4648. https://doi.org/10.1126/sciadv.abd4648
Cerrito, P., Nava, A., Radovčić, D., Borić, D., Cerrito, L., Basdeo, T., Ruggiero, G., Frayer, D. W., Kao, A. P., Bondioli, L., Mancini, L., & Bromage, T. G. (2022). Dental cementum virtual histology of Neanderthal teeth from Krapina (Croatia, 130–120 kyr): An informed estimate of age, sex and adult stressors. Journal of The Royal Society Interface, 19(187), 20210820. https://doi.org/10.1098/rsif.2021.0820
Davies, T. W., Gunz, P., Spoor, F., Alemseged, Z., Gidna, A., Hublin, J.-J., Kimbel, W. H., Kullmer, O., Plummer, W. P., Zanolli, C., & Skinner, M. M. (2024). Dental morphology in Homo habilis and its implications for the evolution of early Homo. Nature Communications, 15(1), Article 1. https://doi.org/10.1038/s41467-023-44375-9
Dean, M. Christopher & Smith, B. Holly. (2009). Growth and Development of the Nariokotome Youth, KNM-WT 15000. In Grine, Frederick E., Fleagle, John G., & Leakey, Richard E. (Eds.), The First Humans: Origin and Early Evolution of the Genus Homo (pp. 101–120). Springer.
Détroit, F., Mijares, A. S., Corny, J., Daver, G., Zanolli, C., Dizon, E., Robles, E., Grün, R., & Piper, P. J. (2019). A new species of Homo from the Late Pleistocene of the Philippines. Nature, 568(7751), 181–186. https://doi.org/10.1038/s41586-019-1067-9
Ingicco, T., Reyes, M. C., de Vos, J., Belarmino, M., Albers, P. C. H., Lipardo, I., Gallet, X., Amano, N., van den Bergh, G. D., Cosalan, A. D., & Bautista, A. (2020). Taphonomy and chronosequence of the 709 ka Kalinga site formation (Luzon Island, Philippines). Scientific Reports, 10(1), 11081. https://doi.org/10.1038/s41598-020-68066-3
Ingicco, T., van den Bergh, G. D., Jago-on, C., Bahain, J.-J., Chacón, M. G., Amano, N., Forestier, H., King, C., Manalo, K., Nomade, S., Pereira, A., Reyes, M. C., Sémah, A.-M., Shao, Q., Voinchet, P., Falguères, C., Albers, P. C. H., Lising, M., Lyras, G., … de Vos, J. (2018). Earliest known hominin activity in the Philippines by 709 thousand years ago. Nature, 557(7704), 233–237. https://doi.org/10.1038/s41586-018-0072-8
Smith, T. M., Tafforeau, P., Cabec, A. L., Bonnin, A., Houssaye, A., Pouech, J., Moggi-Cecchi, J., Manthi, F., Ward, C., Makaremi, M., & Menter, C. G. (2015). Dental Ontogeny in Pliocene and Early Pleistocene Hominins. PLOS ONE, 10(2), e0118118. https://doi.org/10.1371/journal.pone.0118118
van Heteren, A. H., King, A., Berenguer, F., Mijares, A. S., & Détroit, F. (2023). Cementochronology using synchrotron radiation tomography to determine age at death and developmental rate in the holotype of Homo luzonensis (p. 2023.02.13.528294). bioRxiv. https://doi.org/10.1101/2023.02.13.528294
Zanolli, C., Kaifu, Y., Pan, L., Xing, S., Mijares, A. S., Kullmer, O., Schrenk, F., Corny, J., Dizon, E., Robles, E., & Détroit, F. (2022). Further analyses of the structural organization of Homo luzonensis teeth: Evolutionary implications. Journal of Human Evolution, 163, 103124. https://doi.org/10.1016/j.jhevol.2021.103124
John Hawks Newsletter
Join the newsletter to receive the latest updates in your inbox.